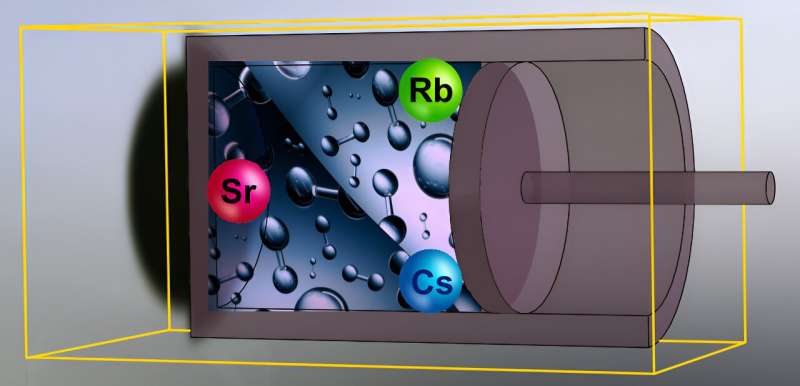
Skoltech scientists and their colleagues from Shubnikov Institute of Crystallography of RAS and research centers in China, Japan, and Italy have discovered a material for chemical storage of hydrogen that can "soak up" four times as much of this hard-to-contain gas as the current top contenders.
Finding efficient ways to confine hydrogen is crucial for integrating this promising energy carrier into the sustainable economy of the future. With proper storage technology, hydrogen could one day fuel high-temperature industrial processes and transportation, and serve for balancing supply and demand on the power grid. The study appears in Advanced Energy Materials.
Hydrogen is expected to play a major role in the future low-carbon economy. It can be produced renewably and consumed to generate electricity or heat via fuel cells or combustion. Some of the areas that stand to gain the most from hydrogen energy are steelmaking, production of glass and cement, and the chemical industry.
International shipping—and transportation and mobility in general—would also benefit. Besides that, hydrogen could help balance the electrical grid by storing excess energy, including the irregular supply from renewable sources.
A major obstacle preventing the widespread adoption of hydrogen power is the lack of safe, sustainable, and economical technology for storing this extremely light (14 times lighter than air), reactive, hard-to-contain, and explosive gas. To accumulate and transport hydrogen in gas cylinders, tubes, cryogenic tanks, and pipelines, it can be compressed or liquefied or perhaps even turned into a solid made up of H2 molecules. But there are multiple pitfalls to this:
- First of all, such processing is very expensive. The compression and refrigeration involved expend the equivalent of about 20%–40% of the total energy ultimately provided by the hydrogen—a very high penalty.
- And even then, hydrogen is so light that despite its being by far the most energy-dense chemical fuel by mass, it still packs only about half as much energy per unit volume as natural gas—compressed or liquefied. This is particularly inconvenient for vehicles.
- Finally, hydrogen is the smallest molecule, so it easily escapes from containers and even permeates into their metal walls, making them brittle and causing cracks and leaks.
"The alternative is chemical storage," says one of the lead authors of the study, Dmitrii Semenok, who holds a Ph.D. in materials science and engineering from Skoltech.
"Certain materials, for example magnesium-nickel and zirconium-vanadium alloys, can store hydrogen in the voids between the metal atoms that make up the crystal structure. Such accumulators provide relatively dense and safe storage and release hydrogen fairly quickly upon demand if heated.
"But while you can tweak the metal alloys in terms of the conditions they require for hydrogen capture and release and how many charge-discharge cycles they withstand, there is a relatively hard limit on how much hydrogen you can cram into those materials: about two hydrogen atoms per one metal atom. And that is the chief figure of merit."
"The compounds we synthesized—cesium heptahydride CsH7 and rubidium nonahydride RbH9—pack as many as seven and nine hydrogen atoms, respectively, per metal atom. And we expect them to be the first such hydrogen-rich materials stable at atmospheric pressure, although the latter requires further confirmation. At any rate, the proportion of hydrogen atoms in these compounds is the highest among all known hydrides, twice as high as in methane CH4," Semenok added.
The principal investigator of the study, Professor Artem R. Oganov, who heads the Material Discovery Laboratory at Skoltech, explained, "We react the hydrogen-rich powder of ammonia borane with either cesium or rubidium. This produces salts known as cesium or rubidium amidoboranes. Heat decomposes those salts into cesium or rubidium monohydrides and lots of hydrogen.
"Since the experiment is run in a cell between two diamonds exerting 100,000 times the atmospheric pressure, the extra hydrogen is forced into the crystal lattice voids, forming cesium heptahydride and rubidium nonahydride—the latter, in two distinct crystal lattice varieties."
According to the researchers, cesium and rubidium are "predestined for this," because of how large their atoms are, resulting in bigger voids in the crystal structure for hydrogen to occupy. The formation of the compounds agrees with the predictions of both the team's simulations and the calculations based on fundamental physical laws.
The presence of the compounds was also confirmed with multiple analytical techniques: X-ray analysis, Raman spectroscopy, and reflection/transmission spectroscopy—the latter was enabled by the contribution of Research Scientist Denis Sannikov from Skoltech's Hybrid Photonics Lab.
The team now intends to repeat the experiment using large-scale hydraulic presses at a lower pressure—about 10,000 atmospheres—to obtain larger amounts of cesium and rubidium polyhydrides and verify that once synthesized, these compounds remain stable even at atmospheric pressure, unlike the other polyhydrides known to date.
More information: Di Zhou et al, Raisins in a Hydrogen Pie: Ultrastable Cesium and Rubidium Polyhydrides, Advanced Energy Materials (2024). DOI: 10.1002/aenm.202400077
Citation: New material for hydrogen storage confines this clean yet troublesome fuel (2024, April 17) retrieved 17 April 2024 from https://techxplore.com/news/2024-04-material-hydrogen-storage-confines-troublesome.html
This document is subject to copyright. Apart from any fair dealing for the purpose of private study or research, no part may be reproduced without the written permission. The content is provided for information purposes only.