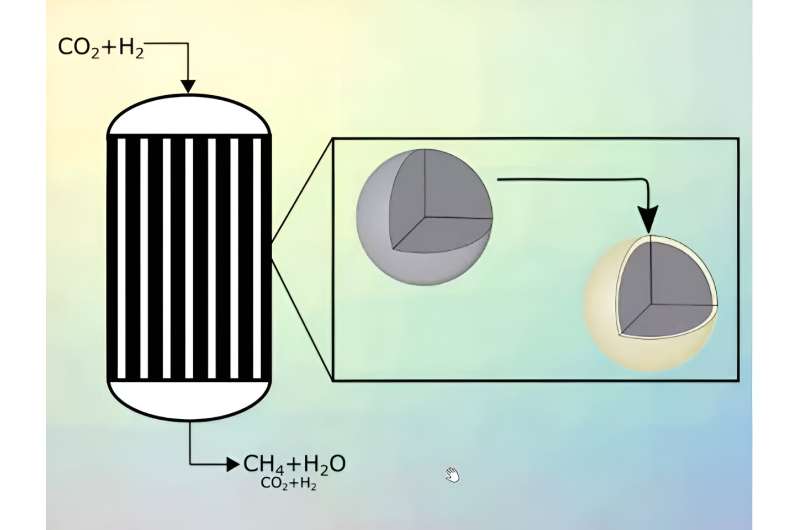
An integral part of the transition to green energy is sensibly utilizing the occasional surplus electricity generated by renewable sources. One possibility is using such surplus energy to produce hydrogen via electrolysis. This green hydrogen can then either serve as an energy carrier itself or be used in further synthesis reactions.
One possible example of such a reaction entails using carbon dioxide to produce methane, which would also help reduce CO2 emissions. The methane can then in turn be used as a replacement for natural gas. However, there are some technical challenges along the way. For example, the reaction releases a lot of heat, which can cause problems.
A research group at the Max Planck Institute for Dynamics of Complex Technical Systems has presented a novel catalytic concept that prevents the reactor from overheating.
The further expansion of renewable energies will increasingly lead to phases in which more electricity is generated than there is a pressing demand for. It is important that we have the capacity to store these surpluses for later use. Battery systems, for example, would be suitable for this purpose. However, it is also possible to use surplus energy for synthesizing chemical substances, which can then serve as energy carriers or raw materials. This concept is also known as Power-to-X. Possible substances include hydrogen, methanol, and ammonia.
Methane is also a sensible storage molecule of green energy. For example, it could replace fossil natural gas, of which it is, after all, the main component. Methane can be generated using carbon dioxide (CO2) via a process known as methanation, during which hydrogen and CO2 react with one another. The necessary hydrogen can be obtained by using the aforementioned energy surplus to electrolyze water. The carbon dioxide in turn could be obtained from industrial waste gases, such as those generated in cement or steel works, power stations, or biofuel factories. This would simultaneously prevent CO2 emissions and retain the carbon within the industrial material cycle.
Catalyst with an active core and an inert shell
There are currently no large-scale methanation facilities that store renewable energy. This can attributed to the technical challenges presented by the process. For example, the reaction of CO2 and hydrogen releases a lot of heat, which can cause the temperature in the reactors to rise sharply. However, they must not climb above 550°C, because the nickel used as a catalyst would then become inactive and the reaction would be brought to a halt.
Consequently, concepts for limiting the reactor temperature are needed. While there are certainly a whole series of technical approaches, many of them are not commercially viable due to the effort and expense involved. So far, no process has made it beyond the pilot plant stage. Researchers at the Max Planck Institute for Dynamics of Complex Technical Systems in Magdeburg have now developed a concept that delivers economically interesting methane yields, while also preventing an unwanted rise in temperature.
The team, led by Kai Sundmacher, Director of the Process Systems Engineering department at Max Planck Institute for Dynamics of Complex Technical Systems in Magdeburg, have developed the concept of core-shell catalyst pellets. "With the development of an active catalytic core and an inert shell, it is possible to limit the reactor temperature, thereby laying the foundations for the large-scale methanation of carbon dioxide," explains Kai Sundmacher. They have published two articles on the concept in the Chemical Engineering Journal.
The key feature of the core-shell approach is the chemically inert porous shell. Molecules that are intended to react with one another must first penetrate this shell, in order to reach the layer of nickel which is present in the core. This is where the methane is formed.
"The diffusion of the reactants through the shell is precisely what slows down the rate of reaction, thereby preventing an excessive increase in temperature," explains Ronny Tobias Zimmermann, a chemical engineer on Sundmacher's team. Zimmermann also explains that, thanks to the properties of the shell, such as its diameter or porosity, you can determine the maximum temperature of the reactor. "The thicker the shell, the lower the maximum possible temperature," Zimmermann concludes. In simple terms: the longer the path the individual molecules take to reach the catalyst, the greater the reduction in speed.
The concept is also compatible with a fluctuating hydrogen supply
The Magdeburg team determined the optimal properties and measurements for the core-shell catalyst pellets and the reactors using computer simulations and subsequent experiments. Following this experimentation, a single approach was arrived at, in which a bundle of 3-meter-long pipes just a few centimeters thick is used as a reactor. The pipes are then filled with core-shell catalyst pellets. These are around 3 millimeters in diameter , with shells that are precisely 0.1 millimeters thick. This equates to roughly double the thickness of a single human hair. For the methanation, a mixture of hydrogen and carbon dioxide is fed through the tubes, which are tempered to around 300°C. The resulting methane gas is collected at the other end of the pipes, cleaned and can then be fed into tanks or into the existing natural gas network.
"Everything has been designed in such a way, that the reactor never exceeds a temperature of 480°C, no matter how much raw material we feed into the reaction," explains Zimmermann. This prevents the nickel from becoming inert. This flexibility and robustness is particularly important with regard to the use of renewable energies, which sometimes supply more, sometimes less surplus electricity. The amount of hydrogen that is produced and can then react with carbon dioxide fluctuates in accordance with the available electricity supply. For process systems engineering, such variations in the quantity of the reactants is generally a significant challenge. Solutions that also deal with these fluctuations are also known as load flexible.
Versatile applications for core-shell catalyst pellets
"The problem can naturally be solved by intermittently storing and steadily retrieving the hydrogen" explains Sundmacher. "Storing hydrogen in this way is however very cost intensive." Consequently, a load-flexible solution of the kind that has now been developed is of great interest. In principle, the Magdeburg team envisage their reactor being utilized to obtain methane in all instances where surplus renewable electricity can be used to supply the necessary hydrogen. This includes both wind parks and larger photovoltaic installations.
The Max Planck researchers noted that their approach is not limited to the reaction of hydrogen with carbon dioxide. Generally speaking, they explain, the concept of tailor-made core-shell catalysts can be applied to all gas reactions which generate high levels of heat, for example, to the reaction of hydrogen and nitrogen to generate ammonia. The Magdeburg Institute is currently also involved in the H2Mare project. Generally speaking, this is focused on the direct use of surplus electricity by offshore wind facilities to synthesize hydrogen. With regards to the possible further uses for the hydrogen obtained, the researchers also investigate the possibility of producing methane and ammonia in reactors containing core-shell catalyst pellets.
In any event, Kai Sundmacher and his team see enormous potential for storing renewable electricity in chemicals and energy carriers. Currently, the main limitation is the amount of available renewable energy. This is something that is nevertheless set to change in future.
More information: Ronny Tobias Zimmermann et al, Core–shell catalyst pellets for effective reaction heat management, Chemical Engineering Journal (2022). DOI: 10.1016/j.cej.2022.140921
Ronny Tobias Zimmermann et al, Load-flexible fixed-bed reactors by multi-period design optimization, Chemical Engineering Journal (2021). DOI: 10.1016/j.cej.2021.130771
Citation: From greenhouse gas to green fuel (2024, January 12) retrieved 12 January 2024 from https://techxplore.com/news/2024-01-greenhouse-gas-green-fuel.html
This document is subject to copyright. Apart from any fair dealing for the purpose of private study or research, no part may be reproduced without the written permission. The content is provided for information purposes only.